Atomic Number:
Atomic Mass: 294
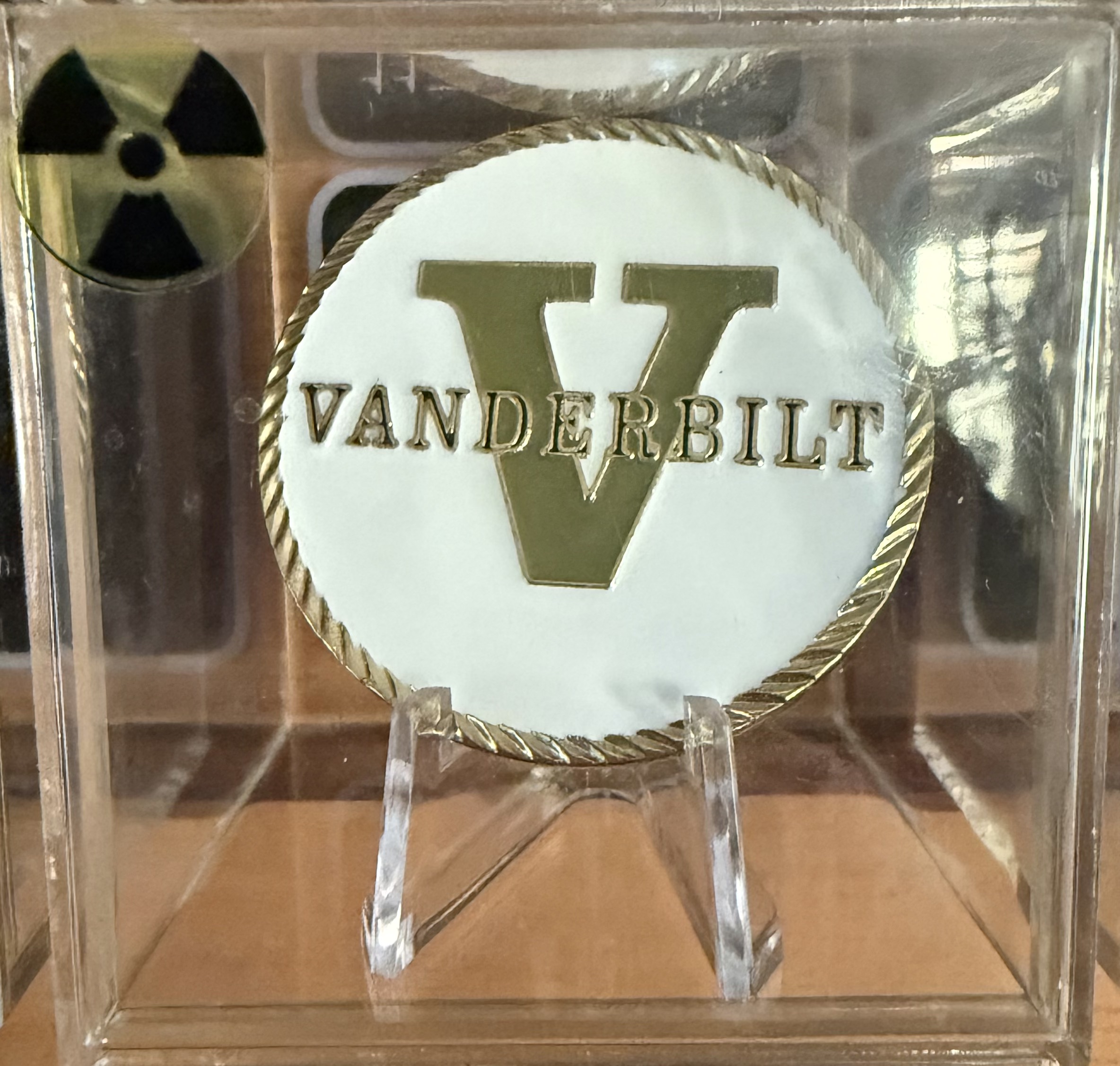
Tennessine, with the atomic number 117, is a synthetic superheavy element that exists only for a fraction of a second, but its discovery marks a major milestone in the field of nuclear chemistry. Named after the U.S. state of Tennessee, in recognition of the contributions of its research institutions, Tennessine’s creation was the result of international collaboration and cutting-edge scientific techniques. Although it has no practical uses due to its extreme instability, the study of Tennessine is critical for advancing our understanding of atomic structure, nuclear forces, and the limits of the periodic table.
In this blog post, we’ll explore the discovery of Tennessine, its properties, and its significance in modern science.
The Discovery of Tennessine
Tennessine (chemical symbol Ts) was first synthesized in 2010 by a collaborative team of scientists from the Joint Institute for Nuclear Research (JINR) in Dubna, Russia, and the Oak Ridge National Laboratory (ORNL) in Tennessee, USA, with support from Lawrence Livermore National Laboratory in California. The team, led by Yuri Oganessian, created Tennessine by bombarding a berkelium-249 target with accelerated calcium-48 ions in a particle accelerator. The fusion reaction resulted in the creation of Tennessine-294, the first isotope of the element, which has a half-life of only a few milliseconds.
The successful synthesis of Tennessine was a significant achievement, adding another superheavy element to the periodic table and advancing the quest to understand the limits of atomic stability. In 2016, the International Union of Pure and Applied Chemistry (IUPAC) officially approved the name Tennessine, in recognition of the state of Tennessee and its contributions to nuclear research, particularly the efforts of Oak Ridge National Laboratory, which provided the berkelium target used in the experiment.
Properties of Tennessine
As a superheavy synthetic element, Tennessine is highly radioactive and decays rapidly, making it challenging to study in detail. Most of what is known about Tennessine comes from theoretical predictions and brief experimental observations, as its short half-life limits the scope of laboratory research. However, its position in Group 17 of the periodic table, known as the halogens, allows scientists to make educated guesses about its behavior by comparing it to lighter elements in the same group, such as fluorine (F), chlorine (Cl), bromine (Br), iodine (I), and astatine (At).
Here are some key properties of Tennessine:
- Atomic Number: 117
- Atomic Mass: [294] (most stable isotope)
- Classification: Halogen (Group 17)
- Radioactivity: All isotopes of Tennessine are highly radioactive, with short half-lives ranging from milliseconds to seconds. The most stable isotope, Tennessine-294, has a half-life of about 78 milliseconds.
- State: Tennessine is predicted to be a solid under standard conditions, although no macroscopic sample has ever been produced due to its instability.
Due to relativistic effects—which occur when electrons in heavy elements move at speeds close to the speed of light—Tennessine is expected to behave somewhat differently from its lighter halogen counterparts. While lighter halogens are known for forming salts and being highly reactive, Tennessine’s large atomic size and relativistic effects may make it less reactive, more metallic, and possibly even chemically inert in some situations. However, these predictions remain largely theoretical due to the difficulty of studying Tennessine in detail.
Modern-Day Uses of Tennessine
Tennessine, like other superheavy elements, has no practical applications due to its extreme instability and short half-life. It decays within milliseconds, making it impossible to use in industrial or technological processes. Instead, Tennessine’s value lies in its role in scientific research, helping scientists explore the boundaries of nuclear stability and atomic structure.
The study of Tennessine provides valuable insights into the forces that hold atomic nuclei together and the behavior of very heavy elements. By investigating Tennessine, researchers hope to refine their understanding of the periodic table’s upper limits and guide the search for new, more stable elements.
Tennessine in Scientific Research
One of the main reasons for studying Tennessine is to investigate the island of stability, a theoretical region of the periodic table where certain superheavy elements are predicted to have significantly longer half-lives. Elements within this island would have nuclear configurations that make them more stable than those currently known, potentially allowing for more detailed studies and practical applications.
Although Tennessine itself is not located within the island of stability, studying its properties helps scientists test their models of nuclear physics and improve their understanding of how atomic nuclei behave at the edge of the periodic table. This research is important for advancing nuclear chemistry and understanding the fundamental forces that govern atomic stability.
Additionally, the study of Tennessine provides insights into relativistic effects, which influence the behavior of electrons in very heavy elements. These effects can cause elements like Tennessine to behave differently from their lighter counterparts, and understanding them helps scientists refine their models of atomic structure.
How Is Tennessine Produced?
Tennessine, like other superheavy elements, is produced through nuclear fusion reactions. In the case of Tennessine, scientists bombarded a berkelium-249 target with calcium-48 ions, creating Tennessine through the fusion of these atomic nuclei. However, due to the complexity of the process and the instability of the element, only a few atoms of Tennessine can be produced at a time.
Once created, Tennessine decays almost immediately, primarily through alpha decay, in which it emits helium nuclei (alpha particles) and transforms into lighter elements. The short half-life of Tennessine makes it challenging to study, and sophisticated detection equipment is required to observe its brief existence and analyze its decay products.
The Future of Tennessine Research
While Tennessine itself may not have practical applications, its discovery represents a major step forward in our understanding of superheavy elements and the structure of atomic nuclei. As researchers continue to develop more advanced particle accelerators and detection methods, they hope to produce larger quantities of Tennessine and other superheavy elements, allowing for more detailed studies of their properties.
Ongoing research into Tennessine also contributes to the broader search for new elements beyond the current limits of the periodic table. By studying Tennessine and similar elements, scientists can refine their understanding of nuclear stability and guide future experiments aimed at discovering elements with longer half-lives and potentially practical uses.
Conclusion
Tennessine, the superheavy element named in honor of Tennessee, is a fascinating addition to the periodic table and a symbol of international collaboration in nuclear science. While it has no practical applications due to its extreme instability, Tennessine plays a crucial role in advancing our understanding of atomic structure, nuclear forces, and the upper limits of the periodic table.
As scientists continue to explore the behavior of superheavy elements like Tennessine, they are pushing the boundaries of nuclear chemistry and gaining new insights into the forces that shape atomic nuclei. The discovery of Tennessine is a significant achievement in the quest to understand the final frontier of the periodic table, and it may one day lead to the discovery of new elements that transform our understanding of the atomic world.
Comments are closed